2D Materials & Quantum Matter
Controlling how materials interact with complex environments enables advances in fields ranging from biological and chemical sensing to artificial photosynthesis. In the WSI, we create hybrid organic/inorganic interfaces, semiconductor/electrolyte junctions, and engineered solid state heterostructures to achieve specific functionality and explore interactions where solid materials meet their surroundings.
Project Modules

2D Magnetic Semiconductors
The chromium chalcogen halides (CrXH) are an emerging family of 2D semiconductors with a direct bandgap, highly anisotropic structural and electronic properties, and robust magnetic order with ordering temperatures up to room temperature. We seek to understand and engineer interactions between the magnetic order in CrXH compounds and the excitons they host. The unique magnetic and optical properties of CrXH materials present unique opportunities to develop spintronic devices with magnetic and optical controllability.
Lorem ipsum dolor sit amet, consectetur adipiscing elit. Morbi sapien mi, venenatis sed velit id, feugiat ultrices lectus. Pellentesque ornare felis sit amet est tristique, a pulvinar nunc finibus. Cras et libero sodales mi elementum tempus. Sed magna nisi, congue at rutrum nec, vulputate at neque. Quisque diam felis, bibendum eget velit et, efficitur euismod mauris. Suspendisse consectetur fermentum ligula sed lacinia. Donec imperdiet purus id rhoncus dictum. Duis et eleifend purus. Donec aliquet accumsan diam. Quisque euismod euismod nibh, sed tempor urna mattis vitae. In nec mi fermentum, sagittis libero sed, rutrum lorem.
Duis at sodales sapien. Donec nec justo dictum, vulputate tortor vitae, placerat dui. Pellentesque cursus ultricies mauris rutrum tristique. Nam sit amet leo ut dui tincidunt pretium eget quis sem. Morbi et nisi tellus. Vestibulum ullamcorper, elit ac sollicitudin blandit, risus risus egestas mauris, eu sagittis dolor lorem et ligula. Duis odio purus, rhoncus vitae nisl at, accumsan egestas sem. Aliquam erat volutpat. Morbi est ipsum, sollicitudin sit amet finibus at, sollicitudin ut purus. Duis varius eget quam a dictum. Cras in eleifend justo. Etiam vel aliquam odio.
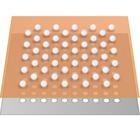
Synthetic superlattices and quantum simulation
Superlattices are artificial, periodic potentials which are used to confined particles and quasiparticles such as atoms, electrons, or excitons. If the trapped quasiparticles are allowed to interact, the resulting many-body state can capture essential behaviors of canonical quantum many-body theories (e.g. the Hubbard model). Such artificial quasiparticle lattices are often called analogue quantum simulators. A bit like a quantum wind tunnel, the quantum simulator serves as an engineerable scale model of a real physical system (electrons or other particles in a real crystalline solid) which allows us to probe complicated many-body physics with a high level of control. Such many-body physics are often hard to describe or predict theoretically, so our quantum simulators provide a route to benchmark leading theories and discover new phases of matter beyond current theoretical predictions.
Our approach to quantum simulation is to create superlattices in 2D semiconductors by engineering an artificial electrostatic potential. This is accomplished by applying a bias voltage between a monolayer semiconductor and a nearby thin graphite layer which has been patterned with a periodic array of holes, generating a periodic potential/electric field profile in the 2D semiconductor layer which can trap charges. The depth of the superlattice potential can be easily tuned, and any lattice geometry can be implemented due to our top-down fabrication approach. We probe the superlattice devices using a combination of optical spectroscopy, relying on the natural sensitivity of the optically-active excitons to the correlated behavior of the charges in the superlattice, complemented by charge transport measurements.
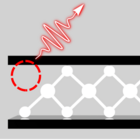
Single-atom circuits
We generate optically active defects in atomically thin 2D materials on a spatial scale of below 10 nm, such that scalable opto/electronics based on individual atomic states seems at reach. We use a helium-ion microscope (HIM) to generate individual defects, e.g. in semiconducting MoS2. Our single-defect technology is principally applicable to the wide range of 2D materials with more than a thousand different materials and is compatible with standard cleanroom manufacturing steps. This combination makes it possible to realize first devices based on single defects, such as gate-switchable single photon emitters and photodetectors or even solar cells based on individual atomic defects. At the same time, fundamental processes, such as the coherent single electron tunneling dynamics and many-body interactions of localized states in a Fermion boson mixture are experimentally accessible.
Collaborators: Jonathan Finley (TUM), Kai Müller (TUM), Nicolas Leitherer-Stenger (DTU), Sivan Refaely-Abramson (Weizmann), Alex Weber-Bargioni (Berkeley)

Excitonic Many-Body States towards Bose-Einstein Condensates
Increasing the interaction strength between quasi-particles in solid-state materials can cause strong correlations, collective phenomena and the transition to macroscopic quantum phases, such as a Bose-Einstein condensate. Heterostructures made of semiconducting 2D materials, such as MoSe2 and WSe2, are ideal systems to realize interacting exciton ensembles, because they provide large exciton binding energies, long photoluminescence lifetimes, as well as a permanent exciton dipole. The latter allows the manipulation of the exciton ensembles, e.g. via electric fields. Recently, we have reported on several signatures regarding photoluminescence intensity, linewidth, as well as spatial and temporal coherences in accordance with the predicted degeneracy of an exciton ensemble at low temperature. The ongoing studies want to explore further predicted phases, such as an excitonic superfluidity and possible condensation phenomena in momentum space.
Collaborators: Ursula Wurstbauer (University of Münster), Andreas Knorr (TU Berlin).